TECHNOLOGY
A Guide to Understanding Keyword Competition in SEO
When it comes to Search Engine Optimisation, keywords are key to getting your website seen. But it’s not just about choosing any keywords — it’s about choosing the right ones. Some keywords are much more influential than others. However, this might be a confusing area for many businesses and individuals. So, here’s our guide that explains keyword competition in detail for your SEO in Melbourne.
What is Keyword Competition?
Ranking high in search results for popular terms is a fierce battle. Many sites vie for top spots, leaving newcomers struggling to break through. Yet hidden gems exist – less sought-after keywords offer easier paths to visibility. While these may draw smaller crowds, they open doors for emerging players. The challenge lies in balancing reach and attainability, navigating the competitive landscape of search engine optimization. Success demands strategic keyword selection, weighing difficulty against potential rewards.
Several factors contribute to keyword competition, including:
– The number of websites already targeting the keyword.
– The domain authority and page authority of competing sites.
– The quality and relevance of the content competing for that keyword.
Why Keyword Competition Matters
Keywords can make or break your SEO strategy. Highly competitive ones demand substantial investment but promise greater traffic. Less contested terms offer quicker gains yet fewer visitors. Assessing competition guides resource allocation and timeline expectations. Will you need months or years to reach Google’s first page? The answer lies in your keyword choices.
A balanced approach, blending high and low-competition keywords, paves the way for enduring SEO success. Carefully weigh your options to optimise both short-term wins and long-term growth.
Factors That Affect Keyword Competition
1. Search Volume
Keywords sought frequently each month have higher search volumes. These popular terms often face fierce competition as websites vie for traffic. Yet exceptions exist. Some niche phrases, though widely searched, encounter less rivalry. Fewer businesses may target these specialized keywords, creating opportunities.
Remember, search volume alone doesn’t dictate competitiveness. Savvy marketers consider both factors when crafting strategies to reach their audience effectively.
2. Relevance to Your Business
The relevance of a keyword to your business or industry can impact how competitive it is. If a keyword is highly relevant to many businesses in a particular industry, competition will naturally be higher. For instance, a broad keyword like “best laptop” is likely to have significant competition, whereas a more specific term like “best laptop for graphic designers under $1000” may have lower competition.
3. DA and PA
Moz’s Domain and Page Authority metrics gauge website and page strength. Higher scores boost ranking potential for competitive keywords. This advantage creates a barrier for emerging sites, hampering their ability to reach top search positions. These influential indicators shape the digital landscape, determining visibility and success in search results.
4. Content Quality
Content quality reigns supreme in keyword battles. Top-ranked sites boast stellar writing, deep insights, and captivating prose. To dethrone them, your content must soar even higher. Google rewards user value above all. Flimsy, copied, or subpar material falters, regardless of keyword prowess. Outshine competitors with substance, not just strategy. Craft content that informs, engages, and elevates the user experience to climb the search rankings.
5. Backlinks
High-quality backlinks boost a website’s competitive edge. These inbound links from other sites shape keyword competition. More backlinks often mean greater authority. Smaller sites struggle to secure links from reputable sources. This challenge intensifies keyword battles. Authoritative backlinks are crucial yet elusive. Their scarcity drives up competition, making keyword dominance harder to achieve.
How to Measure Keyword Competition
1. Google Keyword Planner
Google Keyword Planner, a free Google Ads tool, offers more than just paid search insights. It categorises keyword competition as “Low,” “Medium,” or “High” based on advertiser bidding. Though this metric primarily reflects paid search dynamics, it provides a useful starting point for organic competition analysis.
2. Ahrefs Keyword Difficulty (KD) Score
From 0 to 100, Ahrefs’ Keyword Difficulty score gauges SEO competition. Low scores (0-20) signal easier ranking prospects, while high scores (50+) indicate fierce battles. This popular tool assesses keyword competitiveness by analysing backlink profiles of top-ranking pages. SEO strategists rely on Ahrefs’ KD metric to identify promising opportunities and navigate the complex landscape of search engine optimisation.
3. Moz Keyword Difficulty
Ranking difficulty for keywords is gauged by Moz’s Keyword Difficulty metric. This score weighs factors like top-ranking pages’ authority and their inbound links from unique domains. Moz’s tool also reveals competitors’ domain strength, enabling site-to-site comparisons. These insights help strategise SEO efforts and assess potential ranking challenges.
4. SEMrush Competitive Density
SEMrush’s competitive density score reveals the number of domains vying for paid ad space on specific keywords. Though primarily geared towards paid advertising, this metric offers valuable insights for organic SEO strategies. When combined with SEMrush’s keyword difficulty score – a 0-100 scale – marketers gain a comprehensive view of both paid and organic keyword landscapes.
5. Manual SERP Analysis
One of the best ways to measure keyword competition is through a manual analysis of the SERPs for your target keyword. This gives you a hands-on understanding of what you’re up against. Here’s what the process involves:
– Reviewing the top 10 results.
– Evaluating the domain authority and page authority of the top-ranking websites.
– Checking the content’s quality and the number of backlinks for these pages.
Strategies for Navigating Keyword Competition
1. Target Long-Tail Keywords
Long-tail keywords are specific phrases with lower search volume and competition. For example, instead of “smartphones,” aim for “best smartphones for students under $500.” These keywords often lead to higher sales because they match user intent closely.
2. Create High-Quality, Unique Content
To stand out in a crowded keyword space, your content must surpass existing top ranks. This involves offering detailed information, unique insights, and better-addressing user intent. Moreover, high-quality content naturally draws backlinks, improving your rankings.
3. Build a Strong Backlink Profile
Getting quality backlinks from trusted sites is key to boosting your site’s ranking for competitive keywords. You can earn these backlinks by creating valuable content like research studies, infographics, and expert interviews. Additionally, guest blogging and contacting influencers can also secure you valuable backlinks.
4. Focus on On-Page Optimisation
On-page optimisation involves SEO techniques for your website’s content and structure. Key aspects include placing keywords in headings, meta descriptions, and body content. Additionally, optimising images and internal links is crucial. These steps can enhance your visibility in competitive keyword settings.
5. Leverage Local SEO
If your business targets a specific area, local SEO boosts your regional keyword ranking. Key steps include optimising your Google My Business profile, using location keywords, and earning positive reviews. This strategy particularly benefits small and medium-sized businesses.
Conclusion
If you need better support in understanding keywords, feel free to speak with an expert SEO in Melbourne. We recommend Make My Website, a reliable SEO marketing agency in Melbourne.
TECHNOLOGY
Grey Leather Galaxy Tech 2 Seater Recliner Sleek Comfortable
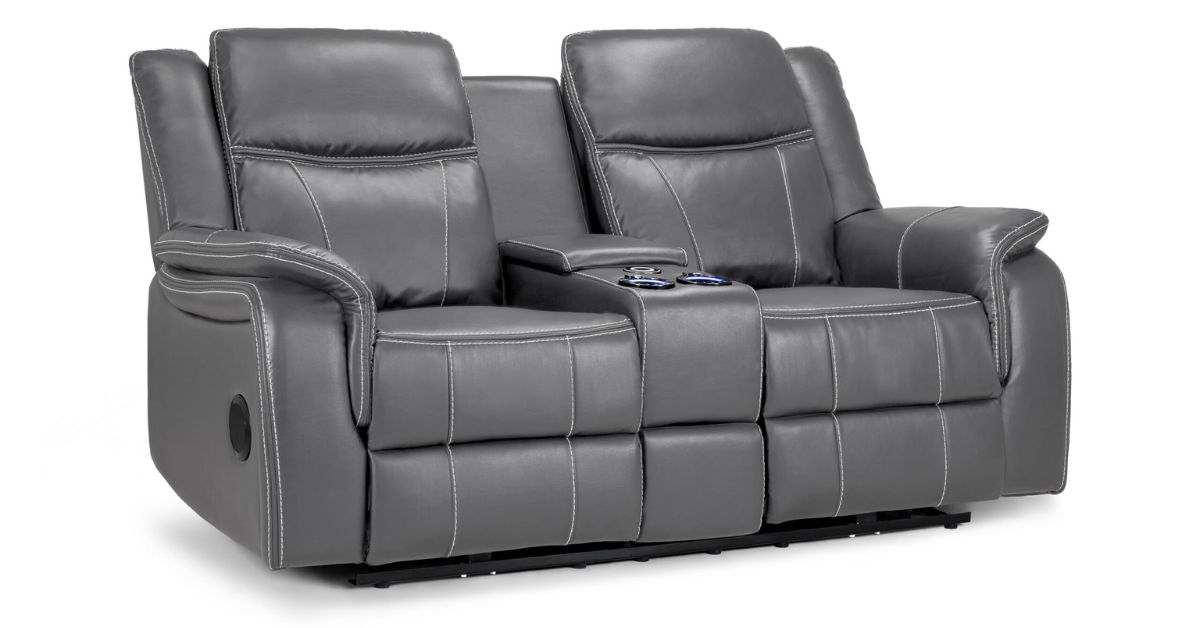
The Grey Leather Galaxy Tech 2 Seater Recliner brings together the fine of each worlds: sleek, cutting-edge design and cutting-edge comfort capabilities. Perfect for those who are seeking for a stability among style, luxurious, and functionality, this recliner promises an enjoy not like every other. Its flexible gray leather-based end makes it a natural healthy for diverse home décor styles, from contemporary to minimalist interiors. Whether you’re unwinding after a protracted day or hosting a relaxed movie night time, the Galaxy Tech Recliner offers the last in rest and comfort.
Design and Aesthetics
One of the first stuff you’ll notice approximately the Grey Leather Galaxy Tech 2 Seater Recliner is its glossy, present day design. The smooth traces, refined proportions, and minimalist structure make a contribution to its cutting-edge enchantment, making it a standout piece in any living room or entertainment space.
The recliner’s gray leather upholstery provides a hint of elegance and class. Grey, being a neutral colour, without problems enhances a huge variety of interior styles and colour schemes. It exudes a subtle, refined luxurious that blends seamlessly with present-day décor, but it stays flexible sufficient to complement extra conventional or eclectic styles. The grey leather additionally boasts a rich texture that feels smooth and snug, offering a premium contact without overwhelming the gap.
In terms of size, the two-seater shape is designed to be compact and space-saving, making it ideal for smaller residing rooms or residences. Its streamlined design ensures it suits with no trouble in relaxed spaces without sacrificing comfort or capability. Whether you’re seeking to grant a current studio or a circle of relatives room, this recliner offers both style and practicality.
Premium Comfort Features
When it involves consolation, the Grey Leather Galaxy Tech 2 Seater Recliner simply excels. The chair is engineered for optimum relaxation, with more than a few features designed to aid your frame’s natural curves and will let you unwind instantly.
First and fundamental, the reclining feature lets both seats tilt back easily the touch of a button, providing you the freedom to adjust your seating function for maximum comfort. Whether you prefer to sit upright for analysing or completely reclined for snoozing, this recliner gives more than one position to fit your choice. The recliner’s mechanism is quiet and smooth, taking into account a continuing transition between distinctive seating positions.
For extra consolation, the recliner is prepared with padded armrests, a luxurious headrest, and lumbar support. These capabilities work together to provide superior cushioning, supplying your body the guide it needs during extended sitting classes. The foam padding is dense but soft, making it the perfect balance of assist and plushness.
The ergonomic design ensures that users can enjoy the recliner for long durations without experiencing soreness. Whether you’re watching TV, analysing an ebook, or surely relaxing, the properly-cushioned, nicely-positioned components of this recliner make it a perfect choice for remaining relaxation.
Built-In Tech Enhancements
What sets the Grey Leather Galaxy Tech 2 Seater Recliner apart from conventional recliners is its incorporation of modern technological functions. As the name suggests, this recliner embraces superior tech functionalities to beautify the user’s enjoyment.
The recliner comes equipped with energy reclining features, managed through a touch-touchy button or faraway. The electricity recline mechanism allows you to modify your seat without difficulty, putting off the need for guide adjustments. This delivered convenience is a recreation-changer for each person who wants to chill without the effort of pulling a lever or pushing back manually.
Another standout feature of the Galaxy Tech Recliner is its USB charging ports. These included ports allow you to effortlessly price your gadgets without needing to get up from your chair. Whether you’re charging your cellphone, tablet, or laptop, the USB ports make certain that you continue to be powered up, making it a perfect chair for folks who like to multitask or work from the comfort of their recliner.
For the ones looking for an introduced touch of luxury, a few models of the Galaxy Tech recliner also come with LED lights and non-compulsory massage/heating functions. The LED lighting create an ambient glow, ideal for evening relaxation. The rubdown and heating features provide soothing relief for sore muscular tissues and offer a spa-like enjoy in the consolation of your own home.
High-Quality Materials
The Grey Leather Galaxy Tech 2 Seater Recliner is crafted using premium substances designed for both sturdiness and luxury. The pinnacle-grain or faux gray leather upholstery (relying on the model) is not most effective visually stunning, but it’s also surprisingly durable. Leather is known for its ability to face up to wear and tear, making this recliner a long-lasting investment. It is proof against fading, stains, and harm, which is specifically beneficial for families or pet owners.
The frame of the recliner is constituted of robust, sturdy substances, ensuring balance and sturdiness. The splendid frame ensures that the recliner can resist regular use without compromising its structural integrity.
In addition, the recliner’s cushions are packed with high-density foam, offering an appropriate level of firmness and softness. This cushioning gives ample assist even as still preserving a luxurious experience, so you’ll never experience too firm or too soft while seated.
One of the benefits of grey leather is its ease of maintenance. Leather is known for being notably low-protection, requiring just occasional cleansing with a leather-safe product to preserve it looking clean. With proper care, the leather will retain its costly appearance for future years.
Space-Saving and Versatility
Despite its costly functions, the Grey Leather Galaxy Tech 2 Seater Recliner does not take up excessive space, making it an ideal choice for folks who need a snug seating option for smaller areas. The compact-seater structure manner it is well-suitable for residences, studios, or small living rooms in which eeveryyinch of space counts.
In addition, the reclining mechanism permits the chair to modify with no need a large quantity of clearance at the back of it. This makes it a exceptional alternative for rooms with confined space, as you don’t have to fear approximately pushing it far faraway from the wall to recline.
For introduced versatility, a few fashions of the Galaxy Tech recliner come with a shared middle console that can residence storage compartments for far-off controls, magazines, or maybe a cup holder. This integrated feature gives extra functionality without compromising on style or ccomfort
User Experience and Reviews
The Grey Leather Galaxy Tech 2 Seater Recliner has obtained rave reviews from users who recognise its comfort, ease of use, and smooth layout. Many customers have highlighted the easy, quiet reclining function, which makes it easy to locate the best seating position without the mechanical noise that may be found in conventional recliners.
The USB charging ports have additionally been praised for adding a contemporary contact, permitting users to live linked and rate their gadgets without interrupting their rest time. The ergonomic guide, such as the padded armrests and headrest, has been a standout function, with many customers noting how cushty the chair is for each brief and extended intervals.
While the recliner’s tech capabilities have garnered effective comments, users have also stated how fashionable the chair appears in their dwelling rooms. The gray leather has an undying appeal that elevates the general aesthetic of any space, making it aworthwhileh addition to houses with contemporary and minimalist décor.
Maintenance and Care Tips
To hold the costly appearance of the Grey Leather Galaxy Tech 2 Seater Recliner, regular cleaning is usually recommended. Use a tender, damp material to wipe away dirt and debris from the surface, and once in a while situation the leather with a leather care product to preserve it soft and supple. Avoid the usage of harsh chemical substances or abrasive cleaning gear, as those can damage the leather-based surface.
For the tech additives, make certain the USB ports and reclining motors are stored free of dirt and particles. It is also an amazing idea to once in a while test the reclining mechanism to make sure easy operation.
Price and Value Proposition
The Grey Leather Galaxy Tech 2 Seater Reclinersofa iss a premium product that offers terrific value formoneyh. While it could be priced higher than conventional recliners, the mixture of glossy layout, integratetechnologyon, and long-lasting materials justifies the price. Compared to other recliners within the marketplace, it stands out for its superior functions and modern enchantment, making it a smart investment for every person looking to beautify their living space.
Conclusion
The Grey Leather Galaxy Tech 2 Seater Recliner strikes the best balance among luxury, comfort, and functionality. Its smooth design, first-rate substances, and superior tech capabilities make it a super choice for modern-day houses. Whether you’re trying to add a touch of sophistication to your living room or in reality need a recliner that offers closing comfort, this version gives you on all fronts. With its smooth-to-use reclining mechanism, incorporated charging ports, and ergonomic support, the Galaxy Tech recliner offers the whole lot you need to relax in style.
TECHNOLOGY
Exploring 185.63.253.2pp: An In-Depth Technical Insight
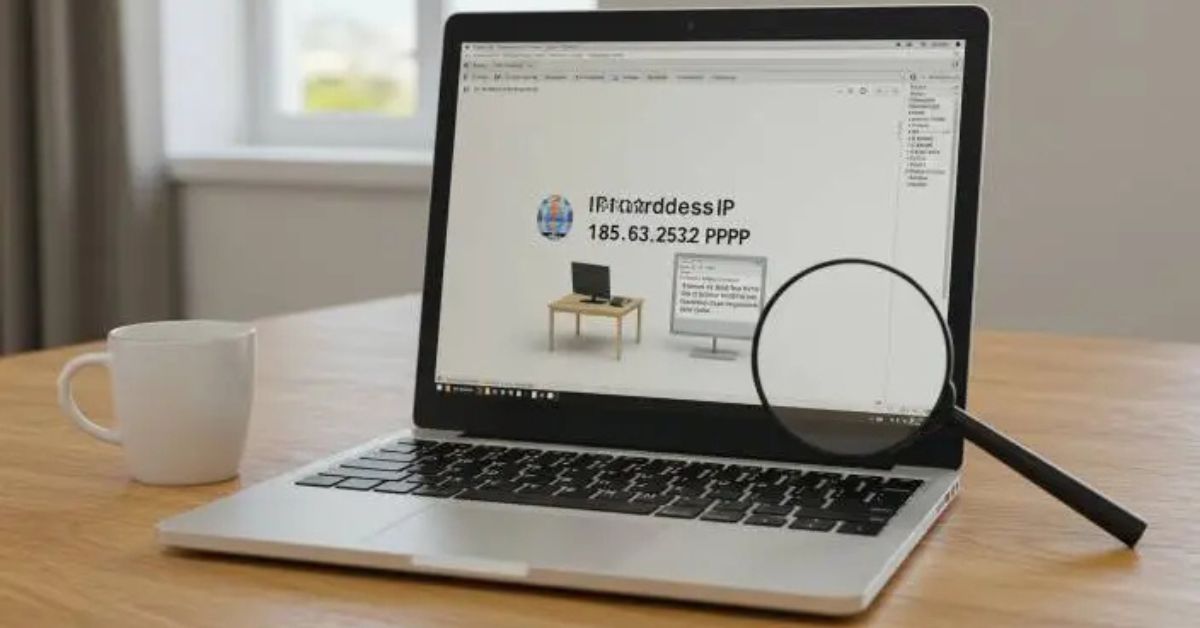
Let’s be real for a second—when you first see something like 185.63.253.2pp, your mind probably jumps to an IP address, right? It looks like it fits the mold. But something feels… off. That “pp” at the end just throws everything out of whack. Is it a mistake? A hidden reference? Or is it part of something more complex? Let’s unpack it.
Understanding the Structure of an IP-like Term
Why “185.63.253.2pp” Doesn’t Follow Conventional IP Standards
Here’s the thing: real IPv4 addresses consist of four groups of numbers, each between 0 and 255, separated by periods. So “185.63.253.2” checks out. But “pp”? That’s not a valid part of an IP address. It breaks the rules—flat out. So this string isn’t an actual IP address. It might be a custom identifier, a placeholder, or even a code error.
Possible Meanings of the Suffix “pp” in Context
Could “pp” stand for something? It might. It could refer to “proxy port,” “peer point,” or just be developer shorthand. In programming and networking, it’s not uncommon to tack on suffixes for internal tracking. So maybe “185.63.253.2pp” is someone’s way of marking a specific resource or endpoint.
Common Misinterpretations and Confusions
Mistaken for a Real IP Address?
Totally. Especially in logs or error messages, where IPs fly by all the time, “185.63.253.2pp” might look legit at first glance. But it won’t resolve in a browser, nor will it ping successfully. So people trying to trace it like a real IP hit a dead end.
Typographical Errors or Symbolic Tags?
Maybe it’s a typo. Or maybe it’s an intentional marker. Think of how “localhost” is used in development. Perhaps “185.63.253.2pp” is a fictional or symbolic stand-in.
Technical Background of IP Formatting
IPv4 vs. IPv6 Standards
IPv4 is what we’re all used to—four numbers, dots between them. IPv6 is longer, uses hexadecimal, and looks totally different. Either way, “pp” doesn’t belong in either format.
Reserved and Private IP Ranges
Some IPs are never used on the public internet. Maybe 185.63.253.2 is one of those reserved ranges, and “pp” just adds context within a local system.
Potential Use Cases of 185.63.253.2pp
Placeholder Text in Code or Config Files
Ever seen “example.com” in documentation? That’s what this might be. A fake IP used to fill space during development.
Identifier in Web-Based Applications
It could be an endpoint reference for an internal tool. Devs sometimes leave cryptic breadcrumbs like this behind.
Reference in Custom Software
If a tool uses non-standard addressing internally, “185.63.253.2pp” could be a legitimate callout—just not a public one.
Is 185.63.253.2pp Dangerous?
Security Implications of Misleading IP Strings
It can be. If someone tricks you into thinking this is a real IP and you interact with it blindly, you could fall into a trap. Fake-looking strings have been used in phishing and spoofing before.
How Cybercriminals Use Similar Tactics
Attackers love confusion. If they use “185.63.253.2pp” in a spoof email or fake DNS entry, users might ignore red flags. Awareness is key.
Investigating 185.63.253.2pp Online
DNS Lookup Results and Tools
Try running this through a DNS tool—nothing meaningful will show. But it helps prove it’s not real, which can put your mind at ease.
WHOIS and IP Tracing Techniques
A WHOIS search might turn up info on “185.63.253.2” alone, but not the full “pp” version. That’s because WHOIS doesn’t recognize or process non-IP suffixes.
Real-World Examples and Reports
Forums and Discussions on the Keyword
A quick search shows that folks are scratching their heads over this one. Most agree it’s either a dev tool relic or a custom label.
False Alarms vs. Real Technical Issues
Some antivirus or firewall logs flag unknown patterns. “185.63.253.2pp” might pop up and trigger false alarms, even if it’s harmless.
Troubleshooting When You Encounter 185.63.253.2pp
Diagnostic Tools for Network Investigation
Use traceroute, ping, and dig. If none return valid results, it’s not a real network path. This is good detective work for any strange string.
Questions to Ask During Debugging
Where did you find it? In code? Logs? Emails? Context is everything when trying to figure out if it’s junk or something meaningful.
Web Hosting and Proxy Considerations
Unusual Strings in Server Logs
Sometimes, log files capture malformed requests. “185.63.253.2pp” could be an artifact of that—garbage data or a malformed entry.
Proxy Config Errors or Redirects
If a proxy misroutes data or logs traffic oddly, strange outputs like this might show up. Not always dangerous, but worth noting.
Could 185.63.253.2pp Be an Internal Reference?
Developer Notation in Enterprise Systems
In internal enterprise networks, you might see strange labeling schemes. “185.63.253.2pp” could just be one team’s way of saying “server 2, private port”.
Misconfigured API Endpoints
A typo in an endpoint URL might spit this out. It might have started life as “185.63.253.2:8080” and got mangled somewhere along the way.
How Developers Should Handle Unknown Strings
Sanitizing Input in Applications
Always filter and validate. If “185.63.253.2pp” shows up where a valid IP should be, code should catch and flag that.
Preventing Log Confusion or Errors
Logs are your eyes into what’s happening behind the curtain. Use clear formats and don’t rely on guesswork. Unknown strings are trouble.
Educational Value of Analyzing Odd IP-like Inputs
Teaching Network Fundamentals Through Errors
Odd cases like this are goldmines for learning. They push you to verify assumptions and research how IPs should look.
Encouraging Digital Literacy and Research
Next time someone asks what “185.63.253.2pp” is, you’ll be the expert. It’s about asking the right questions.
Should You Block 185.63.253.2pp in Firewalls?
Assessing Threat Levels
It’s not a real IP, but if it keeps popping up in logs, that might mean something is misconfigured or spoofing addresses.
Logging and Whitelisting Practices
Keep it logged. Don’t whitelist unknown entries. And use descriptive alerts to make sure admins can act fast if needed.
Future Trends: Rise of Non-Standard Identifiers
Obfuscation and the Internet of Things
IoT devices are everywhere and often use strange naming. Get used to odd strings like “185.63.253.2pp”—you’ll see more like them.
Machine-to-Machine Communication Labels
Machines talk to each other with identifiers we don’t always understand. But analysing strings like this helps us peek into that world.
Conclusion
“185.63.253.2pp” might not mean much on the surface, but it’s a perfect example of how the internet is full of puzzles. Whether it’s a coding quirk, a mislabeled placeholder, or something entirely invented, analysing this odd string helps sharpen your eye for digital detail. Stay curious, stay cautious, and always dig deeper.
TECHNOLOGY
Exploring Mifroom: A New Era of Digital Interaction
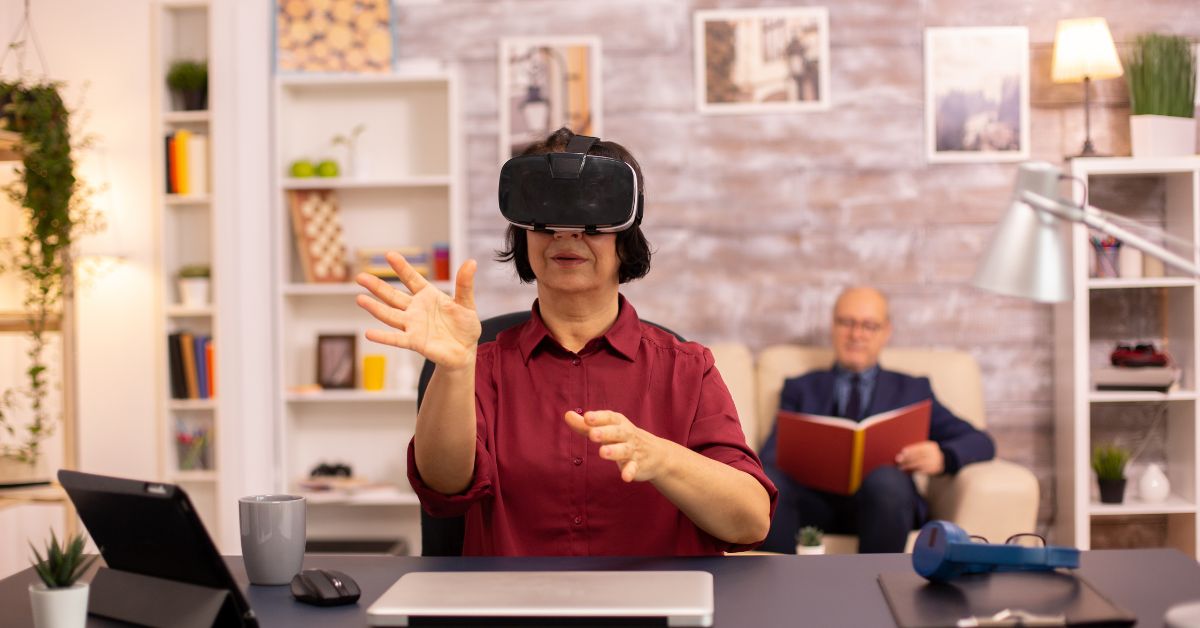
In the rapidly evolving digital landscape, new platforms emerge to address the dynamic needs of users seeking seamless interaction, collaboration, and productivity. One such platform gaining attention is Mifroom. Designed as a multifaceted digital environment, Mifroom aims to unify various aspects of online engagement, offering users a centralized space for communication, project management, and community building.
Understanding the Concept of Mifroom
Mifroom is a versatile digital workspace that integrates tools for collaboration, communication, and organization within a single platform. The name “Mifroom” is a blend of “My” and “Room,” symbolizing a personalized virtual space tailored to individual or team needs. It serves as a hub where users can manage tasks, share files, engage in discussions, and coordinate projects without switching between multiple applications.
Key Features of Mifroom
Unified Dashboard
Mifroom offers a centralized dashboard that provides access to various tools and functionalities. Users can navigate through tasks, calendars, communication channels, and file storage seamlessly, enhancing efficiency and reducing the need for multiple platforms.
Customizable Workspaces
Recognizing the diverse requirements of users, Mifroom allows customization of workspaces to align with specific workflows and preferences. Whether for individual projects or team collaborations, users can tailor their digital environment to optimize productivity.
Collaboration Tools
Mifroom facilitates real-time collaboration through integrated communication tools, including chat functions, video conferencing, and document sharing. These features enable teams to work cohesively, regardless of geographical locations.
Integration Capabilities
To streamline operations, Mifroom supports integration with various third-party applications and services. This interoperability ensures that users can incorporate their preferred tools into the Mifroom ecosystem, maintaining continuity in their workflows.
Security Measures
Understanding the importance of data security, Mifroom implements robust encryption protocols and privacy controls. These measures safeguard user information and ensure compliance with data protection standards.
Applications of Mifroom
Business Collaboration
Mifroom serves as an effective platform for business teams to coordinate projects, manage tasks, and communicate efficiently. Its integrated tools support various aspects of project management, from planning to execution.
Educational Use
Educational institutions and learners can utilize Mifroom for virtual classrooms, resource sharing, and interactive learning experiences. The platform’s collaborative features enhance engagement and facilitate knowledge exchange.
Community Building
Mifroom provides a space for communities and interest groups to connect, share ideas, and organize events. Its user-friendly interface and communication tools foster a sense of belonging and active participation among members.
Advantages of Using Mifroom
Mifroom’s all-in-one approach simplifies digital interactions by consolidating various tools into a single platform. This integration reduces the complexity of managing multiple applications and enhances user productivity. Additionally, its customizable nature allows users to adapt the platform to their specific needs, making it a flexible solution for diverse use cases.
Potential Challenges
While Mifroo’m offers numerous benefits, users may encounter challenges such as a learning curve associated with adopting a new platform. Additionally, the effectiveness of integrations with third-party applications may vary, potentially impacting workflow continuity. Users should assess compatibility with their existing tools and consider training to maximize the platform’s potential.
Future Outlook
As digital collaboration continues to evolve, Mifroo’m is poised to expand its capabilities, potentially incorporating advanced technologies such as artificial intelligence and virtual reality to enhance user experiences. Ongoing development and user feedback will play crucial roles in shaping the platform’s trajectory and ensuring it meets the emerging needs of its user base.
Conclusion
Mifroom represents a significant advancement in digital workspace solutions, offering a comprehensive platform that caters to the multifaceted needs of modern users. By integrating communication, collaboration, and customization, Mifroo’m simplifies digital interactions and fosters productive environments for individuals and teams alike. As it continues to develop, Mifroo’m has the potential to become an indispensable tool in the digital landscape, supporting a wide range of applications across various sectors.
-
TECHNOLOGY1 year ago
Elevating Game Day Eats: A Guide to Crafting Crowd-Pleasing Sliders
-
ENTERTAINMENT1 year ago
wave_of_happy_: Your Ultimate Guide
-
FASHION1 year ago
GPMsign Fashion: Redefining Style with Purpose
-
TECHNOLOGY10 months ago
Trader Joe’s Dayforce: Revolutionizing Workforce Management
-
FOOD1 year ago
Altador Cup Food Court Background: A Culinary Extravaganza Unveiled
-
SPORTS1 year ago
Score Chaser Sporting Clays: A Thrilling Pursuit of Precision
-
NEWS1 year ago
Nyl2 Kemono: Unveiling the World
-
TECHNOLOGY1 year ago
Xatpes: Unveiling the Next Frontier in Technological Advancements